Table of contents
1. OVERVIEW OF Skeletal Muscles and Muscles
Muscle tissue is one of the four types of tissues in the human body, besides epithelium, nervous tissue, and connective tissue. Muscle tissue contributes to the function of movement of the body as well as the movement of organs (contraction of the heart, bowel movements, vasodilation, ...).
Histologically, muscle tissue is divided into two types, skeletal muscle (striated muscle) and smooth muscle (smooth muscle). Under the microscope, in contrast to smooth muscle, which shows a smooth image, striated muscle shows the image of ridges as light-dark bands composed of actin-myosin proteins that are arranged overlappingly, alternating in the ridges. muscle fibers (myofibrils). Anatomically, muscle tissue is divided into skeletal muscle, cardiac muscle, and smooth muscle (Figure 2.1). Cardiac muscle is responsible for the contractile function of the heart. Smooth muscle forms the middle layer of tubular organs (gastrointestinal tract, blood vessels, uterus, etc.) pupil. These activities occur automatically under the control of the sympathetic and parasympathetic nervous systems. Skeletal muscle (skeletal muscle) is a type of skeletal muscle (Figure 2.1), which originates and attaches to the bone, thereby creating joint muscle movements of the body and helping to maintain posture balance. Skeletal muscle contractions are autonomic and are controlled by the somatic nervous system.
Figure 2.1. Anatomical classification of muscle tissue includes (a) Skeletal muscle with long, unbranched muscle fibers (or muscle cells), with peripheral nuclei and striated muscle fibers perpendicular to the length of the muscle fiber. The dark fringes are actually sites where many of the actin and myosin proteins overlap, and conversely, the bright fringes are where there is less overlap. (b) The myocardium is composed of branched muscle fibers but is distinguished by interstitial discs between cardiac muscle cells that assist in conduction of reducing potentials. Cardiac muscle also has filaments containing actin and myosin that overlap and form striated muscle fibers like skeletal muscle. (c) Smooth muscle is distinguished by the absence of striatum and smooth muscle cells are spindle-shaped, flattened cells with a central nucleus, arranged adjacent to each other to create a smooth image compared to striated muscle. first]
Structurally, a skeletal muscle unit (e.g., biceps muscle) is made up of many muscle bundles located together in a muscular sheath. (Figure 2.2). Each muscle bundle consists of many muscle fibers (muscle cells) in a bundle of muscles. Each muscle cell is surrounded by a myofibril and contains several hundred to several thousand filaments composed of the proteins actin and myosin. These proteins are arranged alternately into contractile units (sarcomere). The above-mentioned connective sheaths connect continuously at the muscle tendons with the deep fascia, helping to attach the muscle mass to the bone. Thus, contraction (or relaxation) of a skeletal muscle begins with the shortening (or lengthening) of each unit of contraction. This process is initiated by an action potential across the muscle cell membrane when a nerve signal is transmitted at the neuromuscular synapse (neuromuscular junction).
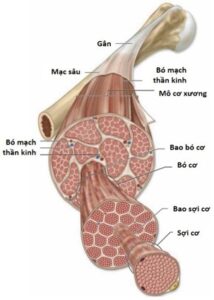
2. STRUCTURE OF ONE MONE CELL
The cell membrane of the muscle fiber is also a phospholipid bilayer, but is divided into grooves – T-tubules or transverse tubules.transverse tubule) – runs deep inside the muscle cell and perpendicular to the muscle fibers (Figure 2.3). It is these grooves that form the interaction between the extracellular fluid and the endoplasmic reticulum of the muscle cells. When an action potential is initiated at the neuromuscular terminal, it is diffused to the T-tubules and stimulates endoplasmic reticulum activity for muscle contraction.
In addition to the myofibrils, the endoplasmic reticulum of muscle cells is a reservoir for intracellular calcium and plays an important role in muscle contraction. The amount of calcium needed to promote the activity of the two proteins actin and myosin is supplied from the endoplasmic reticulum and subsequently, Ca ions.2+ reabsorbed into the endoplasmic reticulum, specifically to the terminal cisternae adjacent to the transverse tubules, to be stored for the next muscle contraction.
Figure 2.3. Structure of the outer muscle cell, consisting of a cell membrane with openings into transverse tubules. These tubules run perpendicular to the length of the muscle fiber and are adjacent to the cisternae terminals of the endoplasmic reticulum and play an important role in the transmission of depolarization signals that increase intracellular calcium [2].
A unit of muscle contraction is calculated from two consecutive “Z” lines containing actin thin filaments and thick myosin filaments. These protein fibers are alternately arranged and slide over each other to induce muscle contraction, using ATP energy from either slow aerobic respiration or fast anaerobic glycolysis. Thin fibers attach the outer end to the Z-disc, while the inner end is free at rest (muscle relaxation). The thick fibers are located in the center of the contractile unit, interspersed with the fine fibers, and are connected to the Z-disc by titin, another protein in the myofibril system. When the muscle contracts, the thick fibers attach to the thin fibers and pull the thin fibers inward, bringing the Z-discs closer (each contraction unit shortens). When the muscle relaxes, the inner end of the thin fiber is released again and the bilateral Z-discs return to their original position (Figure 2.4).
Figure 2.4. (a) A muscle cell contains many muscle fibers. Each filament contains many proteins actin and myosin (also known as thin and thick filaments). The A-band is an area consisting of interlaced thick and thin fibers that produce dark striations on the histological version. Bands I are less overlapping regions, consisting only of alternating fine and titin filaments. (b) The cross section at each position corresponds to the regions and bands on the myofibril. (c) A unit of muscle contraction is bounded by two bilateral Z lines [1]. (d) Contraction or relaxation of a muscle is essentially a shortening or return to its original position (or possibly stretching) of a unit of contraction [3].
3. THE Physiology of Muscles
As mentioned, Skeletal muscle contraction is a deliberate process under the control of the somatic nervous system. Nerve signals are transmitted from the central nervous system by afferent nerves from the anterior horn of the spinal cord to the synapse. A skeletal muscle (e.g., biceps) is arranged into motor units, with each unit consisting of a motor nerve fiber and all the muscle fibers that nerve innervates. (Figure 2.5). Thus, a single motor nerve can transmit to many muscle cells, but a muscle cell is controlled by only one motor nerve. The greater the number of motor units, as well as the number of muscle cells, that are stimulated, the stronger the force of contraction. The stronger the nerve signal from the central nervous system, the more motor units are stimulated. For skeletal muscles performing fine motor, a motor unit contains few muscle cells, while in skeletal muscles performing gross or vigorous movements (biceps, triceps, quadriceps, ...), a motor unit can include hundreds of thousands of muscle cells. Thus, the arrangement of motor units and the level of nerve signals that regulate the force required vary in different activities.
Figure 2.5. Diagram showing somatic nerve innervation starting at the anterior horn of the spinal cord and ending at the neuromuscular synapse on muscle cells. Each motor neuron and its innervated muscle fibers form a motor unit (two motor units are included in the figure) [3].
Chemical transmission at the neuromuscular synapse, through the neurotransmitter acetylcholine, opens the Na channel, causing extracellular Na to flow in and causing depolarization of muscle cells, creating an action potential that helps stimulate muscle contraction (Figure 2.6a). The action potential of the muscle cell is generated when depolarization reaches a threshold of -40 mV and this depends on the concentration of acetylcholine in the postsynaptic cleft. In addition, the action potential is also diffused deep into the muscle cell, to the regions adjacent to the endoplasmic reticulum and myofibril, through the T-tubules. Here, membrane depolarization of the T-tubule activates the receptor. dihydropyridine (DHP receptor), a protein that binds to a calcium release channel on the membrane of the endoplasmic reticulum (also known as the Ryanodine receptor), thereby opening this channel and increasing intracellular calcium. (Figure 2.6b).
Figure 2.6. (a) Neuromuscular junction and the neurotransmitter acetylcholine after being released into the synaptic cleft from the synaptic balls at the nerve terminal [4]. (b) Membrane depolarization in the T tubules activates the Ryanodyin receptor, which releases calcium ions from the endoplasmic reticulum, which increases intracellular calcium and promotes muscle contraction [3].
The binding of calcium ions to troponin protein on the thin filament shifts the tropomyosin protein, revealing the myosin-binding region on the actin protein. (Figure 2.7a). As a result, a bridge is established between the thick and thin fibers, setting the stage for muscle contraction. The higher the concentration of intracellular calcium, the stronger the force of muscle contraction. However, when this concentration reaches the saturation threshold (~10-5M), the maximum force of muscle contraction. For each action potential there is only one release of calcium from the endoplasmic reticulum. Parallel to this process is the reabsorption of intracellular calcium into the endoplasmic reticulum via the calsequestrin pump using ATP.
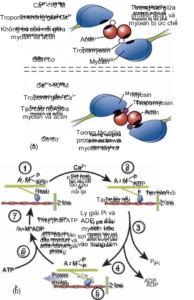
Initially when the muscle is at rest, the myosin head is also bound to ADP and a phosphate group (Pi). (Figure 2.7b). When myosin binds to actin (in a calcium-rich medium), myosin's affinity for ADP and Pi decreases, leading to a structural change in myosin and helping pull actin inward. At this point, the bridge is still held and the muscle contraction is still happening. When ATP binds to the myosin head, actin is released, while the ATP molecule is hydrolyzed to ADP and Pi (the initial state of the myosin head). As nerve signaling continues, intracellular calcium remains high and the muscle shortening limit has not yet been reached, a new cycle continues, myosin reattaches to actin, lysis of ADP and Pi, and pulls the actin filament to slide. inside, and then release actin by ATP. Thus, ATP plays a role in muscle relaxation by (1) releasing actin from the myosin head, (2) activating the calsequestrin pump that reabsorbs intracellular calcium into the endoplasmic reticulum, and (3) activating the calsequestrin pump. Na/K ATPase on the muscle cell membrane to help establish the resting potential.
4. MECHANISM TRANSFORMATION AFTER TRAINING
The muscle fibers in skeletal muscles are divided into two categories, slow-twitch and fast-twitch. The characteristics of these two groups are presented in . (Table 2.1). Any skeletal muscle has enough of both, with varying rates of expression depending on the muscle group (Table 2.2), the function they perform, as well as the training process (Table 2.3).
Table 2.1. Differentiate between slow-twitch and fast-twitch muscles [3]
Slow-twitch muscle |
Fast-twitch, fatigable (Fast-twitch, fatigable) |
• Small size • Use for endurance, high repetition activity (marathon) • The blood system is denser • More mitochondria, more aerobic respiration resulting in more ATP and longer muscle use • Having more myoglobin results in a more prominent muscle red color |
• Twice the size • Suitable when a strong force is needed in a short time (starting when racing, weight training) • Less vascular system and less aerobic respiration • Fewer mitochondria but more glycolytic enzymes for faster ATP generation and short-term use • Less myoglobin and more endoplasmic reticulum → increase intracellular calcium faster |
Table 2.2. Prevalence of slow-twitch muscle expression in some skeletal muscles [5]
Muscle |
Rate of expression of slow muscle contraction |
Muscle group at the joint pan (superspinous muscle, subspinal muscle, small round muscle, subscapular muscle) |
Average 44% |
deltoid muscle |
47% – 74% |
Big butt muscles |
52% – 60% |
Thigh rectus muscle |
from 30% to less than 50% |
The muscle group includes the extrinsic broad muscles, the medial broad muscles, and the inner broad muscles |
44% – 64% |
Muscle group includes semi-tendon, semi-membranous muscle, and biceps muscle |
44% – 67% |
Abdominal muscles |
44% – 65% |
Sandal muscle |
~ 70% |
multifidus muscle |
~ 65% |
Table 2.3. Expression rate of muscle fiber group by type of sport [3]
Type |
Expression rate of rapid muscle contraction (%) |
Expression rate of slow contractile muscle (%) |
Running a marathon |
18 |
82 |
Swimming |
26 |
74 |
Weightlifting |
55 |
45 |
Sprint |
63 |
37 |
Dance |
63 |
37 |
In endurance training (endurance training), exercise with mild resistance over long periods of time, skeletal muscle adapts by increasing mitochondrial activity (and possibly mitochondrial count) and increasing perfusion density to ensure an adequate supply of oxygen for metabolism. aerobically, generating enough ATP to sustain endurance for a long time. Endurance exercises that use large muscle groups at a steady, rhythmic rhythm for at least 30 minutes (brisk walking, jogging, biking, swimming, etc.).
Resistance training (strength training or high-resistance training) increases muscle strength by altering neuromuscular response and muscle hypertrophy. This phenomenon occurs in about 2-3 months of practice. At that time, the nerve signal will mobilize more motor units to increase muscle strength. Anaerobic metabolic exercises are high-intensity exercises that take place in a short period of time, such as sprinting, weightlifting, etc. Thus, the muscle phenotype will also be changed to suit the type. movement, in which case rapid muscle contraction will be more expressed [6].
Muscle hypertrophy (muscle hypertrophy) is an increase in muscle mass, i.e. an increase in the amount of actin and myosin proteins in muscle cells in response to high levels of use or muscle contraction with heavy loads (eg, lifting weights). Muscle hypertrophy also increases the synthesis of metabolic enzymes for glycolysis, helping to generate rapid energy during short-term muscle contractions.
Muscles can also change length in response to stretching or contracting. This is also referred to as muscle hypertrophy, because when the muscle is stretched beyond its limit, new contractile units are formed and added to either side of the myofibril. In contrast, when the muscle is contracted, the functional units at the ends of the myofibril are destroyed. These processes occur to rebuild the muscle in a way that is suitable for the action to which the muscle responds.
An increase in the number of muscle fibers (fibers hyperplasia) can also occur in extreme cases of exercise. The essence of this process is the splitting of the hypertrophied muscle fibers. However, this phenomenon is rare and even if it does, the number of muscle cells increases by only a few percent [3].
5. MECHANISM TRANSFORMATION AFTER TREATMENT
The muscle response after injury consists of three phases [7]. Within a few days of injury, stage 1 is the result of damage to muscle tissue, causing caseation and muscle degeneration
Parallel to that is the inflammatory response and the infiltration of immune cells. The inflammatory response after about 2-4 days will be relieved by type II macrophages, which support muscle healing and subsequent scarring. During this time, the goal of physical therapy is to support inflammation and wound healing. Rest and avoid heavy exercise in order not to further damage the muscle tissue and surrounding soft tissues [5]. One mechanical effect that has been mentioned as beneficial during this period is massage therapy, which has been shown to have anti-inflammatory and mitochondria-forming properties that help damaged cells heal faster [8].
Within 5 days to 2 weeks after injury, phase 2 is the process of repairing and rebuilding new muscle tissue. These satellite cell (satellite cells) are adult stem cells that proliferate and differentiate into new muscle fibers. In addition, fibrous tissue structures are also formed to replace damaged tissues, but are still fragile and weak. This is the time when occupational therapy can be applied to help patients recover motor functions early; but exercises should be designed to the patient's tolerance level, painless, and monitored to avoid damage to new, weak tissue structures [5]. In addition, in vitro experiments have also shown the role of mechanical stretching on the activation of satellite cells [9].
The final stage is the remodeling of muscle tissue, namely the maturation of newly differentiated muscle cells, the restoration of the nervous system and the vascular network. Fibrosis also occurs more and the resulting fibrous tissue has better tension and bearing capacity during this time. Growth factors (growth factor), i.e. IGF-1, HGF, VEGF, FGF, TGF-β1, PDGF, play important roles in this time interval. Increasing the intensity of exercises at this stage is necessary to help patients optimize their fitness Rehabilitation.
Amyotrophic lateral sclerosis (muscle atrophy) is a decrease in muscle mass or a decrease in the amount of actin and myosin proteins when the muscle is not used for a long period of time (several weeks), e.g. when the muscle loses its nerve supply or when the patient suffers from motionless for any reason. In addition, muscle atrophy is also seen in the elderly (sarcopenia). However, this process is still dependent on the level of exercise of the individual and does not occur equally for all muscle groups [10]. Several studies have shown that immobility-induced atrophy affects slow-twitch muscles more than fast-twitch muscles, as well as extensors more than adductors [11]. These proteins are degraded by the ubiquitin-proteosome pathway, and degenerated muscle cells are replaced by fibrous or adipose tissue at a late stage. Thus, the contractile function of skeletal muscles was not possible before. Furthermore, the fibrous tissue in these “atrophic muscles” tends to shorten and stretching methods in physical therapy should be used to avoid muscle contractures.contracture) [3].
6. PRINCIPLES OF SAFE AND EFFECTIVE EXERCISE
The design of occupational therapy and general training activities should be tailored to each individual as each person has a different tolerance and response level. Factors such as age, gender, body shape as well as injury history should be considered in order to design exercises with appropriate difficulty and nature for each patient.
The difficulty of the exercises needs to be higher than the threshold familiar to the practitioner to promote the response of muscles and soft tissues to therapeutic movement. However, this overshoot should be done at the right time when the injury is sufficiently healed and capable of withstanding the load of exercise and can be achieved by increasing the intensity, duration, or frequency of exercise. gradually. Some other possible approaches include increasing difficulty, reducing the number of breaks, increasing repetition, increasing resistance.
Specifically, the period immediately after the injury, when tissues are damaged and the inflammatory process is maximal, rest as well as the application of physiotherapeutic agents (see chapter 2 of this article). Physical agents used in therapy) to reduce pain, anti-inflammatory, increase vasodilation, support metabolism and promote healing should be focused. Then, when the damaged area is replaced with newly differentiated muscle and fibrous tissue, therapeutic exercises can be used to restore motion early and avoid contractures or stiffness, but It is necessary to ensure a moderate level of pain, no pain and the right tolerance of the patient, in order to avoid affecting the weak new tissues. Gradual increases in exercise intensity, quantity, or intensity can be performed over the next period as the muscle tissue matures and the fibrous tissue becomes more resistant. Warming up and maintaining the right intensity play an important role in preventing secondary injury or muscle soreness from exercise.
Based on the physiological response of muscles to different types of exercise, it can be seen that the percentage of slow-twitch muscle expression is higher after endurance training exercises (low resistance, high repetition, duration of exercise). long and targeting aerobic respiration). In contrast, strength training exercises (training with high resistance, short duration) will promote the expression of fast-twitch muscle fibers.